Contents
- 1 1. Introduction to 3D Printing 101:
- 2 2. How Does 3D Printing Work?
- 3 3. Types of 3D Printing Technologies:
- 4 4. Materials Used in 3D Printing:
- 5 5. The Importance of 3D Modeling:
- 6 6. Software for 3D Printing:
- 7 7. Preparing Your Model for Printing:
- 8 8. Operating a 3D Printer:
- 9 9. Common Challenges and Troubleshooting:
- 10 10. Applications of 3D Printing:
- 11 11. Future Trends in 3D Printing:
- 12 12. Conclusion:
1. Introduction to 3D Printing 101:
Welcome to the realm of 3D printing 101, where imagination takes shape layer by layer, and the boundaries of creation are limited only by your creativity. In this introductory section, we’ll embark on a journey to explore the essence of 3D printing, uncovering its profound impact on industries, hobbies, and the way we perceive manufacturing.
What is 3D Printing?
At its core, 3D printing, also known as additive manufacturing, is a process of creating three-dimensional objects from digital models. Unlike traditional subtractive manufacturing methods, which involve cutting or drilling away material from a solid block, 3D printing builds objects layer by layer, adding material precisely where it’s needed. This revolutionary approach enables the fabrication of intricate designs and complex geometries with unparalleled efficiency and precision.
Significance of 3D Printing:
The advent of 3D printing has heralded a new era of democratized manufacturing, empowering individuals and businesses alike to bring their ideas to life with unprecedented ease and speed. From rapid prototyping and customized production to on-demand manufacturing and medical advancements, the applications of 3D printing span a vast spectrum of industries and disciplines.
History and Evolution:
Although the concept of additive manufacturing dates back several decades, it wasn’t until the 1980s that the first functional 3D printing technology, known as stereolithography (SLA), was developed. Since then, 3D printing has evolved rapidly, with a myriad of techniques and materials emerging to cater to diverse needs and applications. Today, 3D printing has permeated various sectors, from aerospace and automotive to healthcare and consumer goods, revolutionizing the way we design, produce, and interact with objects.
Impact on Society:
The democratization of manufacturing brought about by 3D printing has profound implications for society at large. It fosters innovation by lowering the barriers to entry for entrepreneurs and small businesses, allowing them to compete on a global scale. Moreover, 3D printing promotes sustainability by enabling localized production, reducing waste, and optimizing supply chains. In the realm of healthcare, it facilitates personalized treatments and prosthetics, offering newfound hope to patients around the world.
2. How Does 3D Printing Work?
Embarking on a journey into the realm of 3D printing unveils a fascinating world where digital designs materialize into physical objects with remarkable precision and intricacy. In this section, we delve into the underlying mechanisms of additive manufacturing, exploring the step-by-step process through which 3D printers bring imagination to life.
Principles of Additive Manufacturing:
At the heart of 3D printing lies the principle of additive manufacturing, a process that builds objects layer by layer from the bottom up. Unlike traditional manufacturing methods that rely on subtractive techniques, such as cutting or molding, additive manufacturing adds material gradually, precisely depositing it according to a digital blueprint. This approach enables the creation of intricate geometries and complex structures with unparalleled accuracy and efficiency.
Layer-by-Layer Construction:
The foundation of 3D printing lies in its layer-by-layer construction methodology. Each layer of the object is meticulously crafted by the 3D printer, starting from the bottom and progressing upwards until the final form is realized. This layering process allows for the fabrication of objects with intricate internal features and customized properties, making 3D printing an invaluable tool across a myriad of industries and applications.
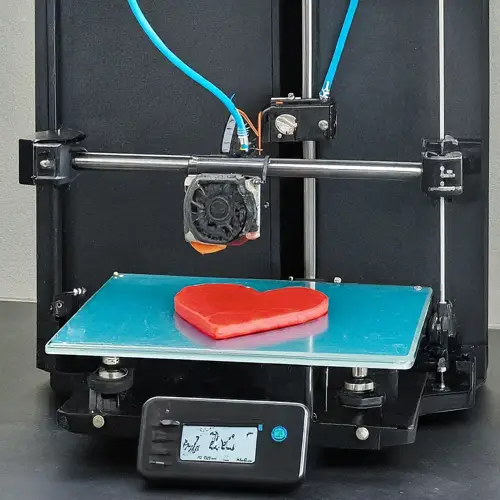
3. Types of 3D Printing Technologies:
In the realm of 3D printing, innovation knows no bounds, with a diverse array of technologies continually pushing the boundaries of what’s possible. From filament-based extrusion to laser-powered sintering, each 3D printing technology brings its unique approach to additive manufacturing, catering to a broad spectrum of applications and requirements. Let’s delve into the fascinating world of 3D printing technologies, exploring their mechanisms, materials, and applications.
Fused Deposition Modeling (FDM):
Fused Deposition Modeling (FDM), also known as Fused Filament Fabrication (FFF), is one of the most widely used 3D printing technologies today. In FDM, thermoplastic filaments are melted and extruded through a heated nozzle onto a build platform, where they solidify layer by layer to form the desired object. This layer-by-layer deposition process allows for the creation of robust, functional prototypes, end-use parts, and intricate models with ease. FDM’s affordability, versatility, and accessibility have made it a favorite among hobbyists, educators, and small businesses seeking to bring their ideas to life.
Stereolithography (SLA):
Stereolithography (SLA) revolutionized the world of 3D printing with its ability to produce high-resolution, intricate parts with exceptional surface finish and detail. In SLA, a vat of liquid photopolymer resin is selectively cured by a UV laser, solidifying it layer by layer according to the digital model. This precise curing process allows for the creation of complex geometries, delicate features, and smooth surfaces that are ideal for applications requiring fine detail and aesthetic quality. From jewelry design and dental prosthetics to architectural models and custom figurines, SLA has found widespread use in industries that demand precision and craftsmanship.
Selective Laser Sintering (SLS):
Selective Laser Sintering (SLS) offers unparalleled versatility and material freedom by utilizing a high-powered laser to sinter powdered materials, such as plastics, metals, and ceramics, into solid objects. In SLS, a thin layer of powdered material is spread across the build platform, and a laser selectively fuses the particles together, layer by layer, according to the digital model. This process allows for the creation of robust, functional prototypes, end-use parts, and complex assemblies with minimal post-processing. SLS is particularly well-suited for applications requiring high strength, durability, and material diversity, such as aerospace components, automotive parts, and medical implants.
Digital Light Processing (DLP):
Digital Light Processing (DLP) 3D printing shares similarities with SLA but utilizes a digital light projector to cure entire layers of photopolymer resin simultaneously. In DLP, the digital model is projected onto the surface of the resin vat, where it is cured by UV light, layer by layer, to form the final object. This rapid curing process enables faster print times and higher throughput compared to traditional SLA, making DLP well-suited for producing large volumes of small, high-detail parts with exceptional accuracy and resolution. DLP finds applications in a wide range of industries, including jewelry manufacturing, dental restoration, and consumer electronics.
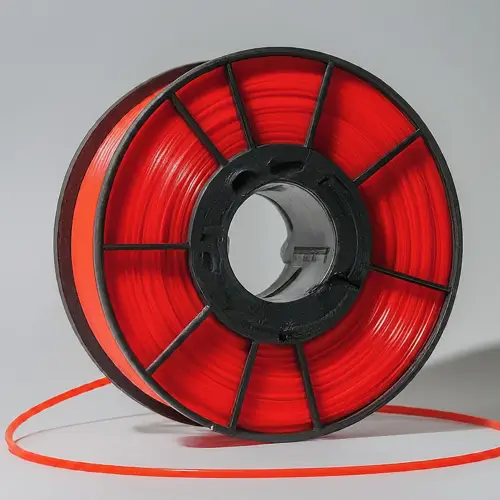
4. Materials Used in 3D Printing:
The versatility and potential of 3D printing extend far beyond its innovative technologies to encompass a vast array of materials, each offering unique properties and applications. From thermoplastics and resins to metals and ceramics, the materials used in 3D printing play a pivotal role in shaping the outcome of the printing process and the characteristics of the final object. In this section, we’ll explore the diverse range of materials utilized in additive manufacturing, shedding light on their properties, suitability, and potential applications.
Thermoplastics:
Thermoplastics represent the most common and widely used material category in 3D printing, owing to their affordability, versatility, and ease of use. Popular thermoplastic filaments include:
- PLA (Polylactic Acid): Renowned for its biodegradability and ease of printing, PLA is a popular choice for beginners and hobbyists. It offers excellent dimensional accuracy and minimal warping, making it ideal for prototyping, educational projects, and decorative items.
- ABS (Acrylonitrile Butadiene Styrene): Known for its durability, impact resistance, and heat tolerance, ABS is favored for functional prototypes, automotive parts, and consumer products. It exhibits superior mechanical properties compared to PLA but requires a heated print bed to minimize warping.
- PETG (Polyethylene Terephthalate Glycol): Combining the best of both PLA and ABS, PETG offers high strength, flexibility, and chemical resistance. It’s suitable for a wide range of applications, including mechanical parts, food containers, and medical devices.
Resins:
Resin-based 3D printing technologies, such as SLA and DLP, utilize liquid photopolymer resins that cure under UV light to form solid objects. Resins come in various formulations tailored to specific applications:
- Standard Resins: Ideal for general-purpose prototyping and modeling, standard resins offer a balance of detail, strength, and surface finish. They are suitable for applications requiring high resolution and intricate geometries, such as jewelry, miniatures, and figurines.
- Engineering Resins: Engineered resins are formulated to meet the demands of functional prototyping and end-use parts in specific industries. They exhibit enhanced mechanical properties, thermal stability, and chemical resistance, making them suitable for applications in aerospace, automotive, and healthcare.
Metals:
Metal 3D printing technologies, such as SLS and Direct Metal Laser Sintering (DMLS), enable the fabrication of high-strength, precision-engineered components from metal powders. Common metal materials used in 3D printing include:
- Stainless Steel: Stainless steel offers excellent corrosion resistance, mechanical strength, and machinability, making it suitable for a wide range of industrial applications, including automotive, aerospace, and medical implants.
- Titanium: Renowned for its exceptional strength-to-weight ratio, biocompatibility, and corrosion resistance, titanium is prized for producing lightweight, high-performance components in aerospace, medical, and defense industries.
- Aluminum: Aluminum alloys combine lightweight with excellent mechanical properties, thermal conductivity, and machinability, making them ideal for automotive parts, consumer electronics, and aerospace components.
Ceramics:
Ceramic 3D printing technologies, such as binder jetting and stereolithography, enable the fabrication of complex ceramic parts with precise geometries and fine details. Ceramic materials offer unique properties, including high temperature resistance, electrical insulation, and biocompatibility, making them suitable for diverse applications:
- Alumina (Aluminum Oxide): Alumina ceramics exhibit exceptional hardness, wear resistance, and thermal stability, making them ideal for engineering components, cutting tools, and biomedical implants.
- Zirconia (Zirconium Oxide): Zirconia ceramics offer superior strength, toughness, and biocompatibility, making them suitable for dental prosthetics, medical implants, and structural components in harsh environments.
- Silicon Carbide: Silicon carbide ceramics combine high hardness, thermal conductivity, and chemical inertness, making them ideal for abrasive tools, refractory linings, and aerospace applications.
5. The Importance of 3D Modeling:
In the realm of 3D printing, where imagination meets reality, the role of 3D modeling cannot be overstated. It serves as the bridge between concept and creation, transforming abstract ideas into tangible objects with precision and efficiency. In this section, we delve into the significance of 3D modeling in additive manufacturing, exploring its essential role in the design process, its impact on innovation, and its transformative potential across industries.
Visualization and Conceptualization:
At the heart of 3D modeling lies the ability to visualize and conceptualize ideas in three-dimensional space. Unlike traditional two-dimensional sketches or drawings, which can sometimes lack depth and detail, 3D modeling allows designers and engineers to explore their concepts from every angle, gaining a comprehensive understanding of form, proportion, and scale. This spatial visualization not only enhances creativity and ideation but also facilitates communication and collaboration, enabling stakeholders to share and refine ideas more effectively.
Precision and Accuracy:
One of the primary advantages of 3D modeling is its ability to achieve unparalleled precision and accuracy in design. By leveraging advanced software tools and techniques, designers can create digital models with exact dimensions, tolerances, and specifications, ensuring that the final product meets the desired requirements. This level of precision is particularly crucial in industries where accuracy is paramount, such as aerospace, automotive, and medical device manufacturing, where even minor deviations can have significant implications for performance and safety.
Iterative Design and Prototyping:
3D modeling empowers designers to iterate rapidly through multiple design iterations, refining and improving their concepts with ease. By simulating real-world conditions and testing various configurations virtually, designers can identify potential issues early in the design process, saving time and resources down the line. Moreover, 3D modeling facilitates the creation of prototypes and mock-ups that closely resemble the final product, allowing stakeholders to evaluate design aesthetics, functionality, and ergonomics before committing to mass production.
Customization and Personalization:
In an era of mass customization and personalization, 3D modeling opens up a world of possibilities for creating bespoke, tailored solutions to individual needs and preferences. Whether it’s custom-fit prosthetics, personalized jewelry, or unique consumer products, 3D modeling enables designers to cater to diverse tastes and requirements, unleashing a new level of creativity and innovation in product design. This ability to tailor designs to specific users not only enhances customer satisfaction but also drives brand loyalty and differentiation in the marketplace.
Collaboration and Accessibility:
One of the most significant advantages of 3D modeling is its accessibility and ease of collaboration. With the proliferation of intuitive, user-friendly CAD (Computer-Aided Design) software tools, designers of all skill levels can create sophisticated 3D models without extensive training or expertise. Moreover, cloud-based collaboration platforms and version control systems enable teams to collaborate seamlessly on shared projects, regardless of their geographic location or time zone. This democratization of design tools and collaboration fosters innovation and creativity, empowering individuals and teams to bring their ideas to life more efficiently and effectively than ever before.
6. Software for 3D Printing:
In the intricate world of 3D printing, where imagination takes shape layer by layer, software plays a pivotal role in transforming digital designs into physical objects. From creating intricate models to slicing them into printable layers, a plethora of software tools cater to every stage of the 3D printing workflow. In this section, we delve into the diverse array of software solutions available for 3D printing, exploring their features, functionalities, and applications.
3D Modeling Software:
At the heart of the 3D printing process lies 3D modeling software, which enables designers and engineers to create digital models of their designs with precision and detail. From industry-standard CAD (Computer-Aided Design) software like Autodesk Fusion 360, SolidWorks, and Rhino 3D to open-source alternatives like Blender and FreeCAD, a myriad of tools cater to diverse needs and skill levels. These software packages provide powerful features such as parametric modeling, surface modeling, and sculpting, allowing users to bring their ideas to life with unparalleled flexibility and creativity.
Slicing Software:
Once a 3D model is created, it must be sliced into printable layers before it can be sent to a 3D printer. Slicing software, such as Ultimaker Cura, Simplify3D, and PrusaSlicer, converts the digital model into a series of instructions (G-code) that the printer can understand. These tools offer a range of features, including customizable print settings, support generation, and print preview, allowing users to optimize their prints for quality, speed, and material usage. Moreover, slicing software supports a wide range of file formats and printers, ensuring compatibility with various hardware configurations and workflows.
Mesh Repair Software:
In some cases, 3D models may contain errors or imperfections that can interfere with the printing process. Mesh repair software, such as Netfabb and Meshmixer, helps identify and fix issues such as non-manifold geometry, holes, and intersecting faces, ensuring that the model is watertight and printable. These tools offer automated repair algorithms, manual editing tools, and visualization features to help users identify and resolve potential problems before printing.
Simulation and Analysis Software:
Simulation and analysis software, such as Autodesk Simulation Mechanical and ANSYS Workbench, enables users to predict and optimize the performance of their 3D-printed parts under various conditions. These tools use finite element analysis (FEA) and computational fluid dynamics (CFD) techniques to simulate factors such as stress, heat transfer, and fluid flow, helping users identify potential design flaws and optimize their designs for strength, durability, and efficiency.
Collaboration and Version Control Software:
In collaborative design environments, collaboration and version control software play a crucial role in managing and tracking changes to 3D models across distributed teams. Platforms such as GrabCAD Workbench and Autodesk Vault provide centralized repositories for storing, sharing, and reviewing 3D models, as well as versioning and revision control features to track changes and ensure data integrity. Moreover, these tools facilitate real-time collaboration, allowing team members to communicate, annotate, and iterate on designs seamlessly.
7. Preparing Your Model for Printing:
Before unleashing the power of your 3D printer, it’s crucial to ensure that your digital model is properly prepared for the manufacturing process. In this section, we delve into the essential steps and considerations involved in preparing your model for printing, from optimizing design for manufacturability to configuring print settings for optimal results.
Design Optimization:
Begin by assessing your 3D model for potential design flaws or areas that may pose challenges during printing. Ensure that the model is watertight, meaning there are no gaps or holes in the geometry that could cause printing errors. Address any overhangs or unsupported features that may require additional support structures to prevent sagging or deformation during printing. Additionally, consider optimizing the design for manufacturability by minimizing overhangs, reducing material usage, and optimizing orientation for strength and stability.
Support Generation:
For models with overhangs or complex geometries that require additional support during printing, generate support structures using slicing software. These support structures provide temporary scaffolding to prevent sagging or collapsing of overhanging features during printing. Configure support settings such as support density, angle threshold, and interface layers to balance the need for support with ease of removal and surface finish. Be mindful of where support structures are placed to minimize their impact on the final part’s aesthetics and functionality.
Orientation and Scaling:
Optimize the orientation and scaling of your model to achieve the desired balance between print quality, strength, and material usage. Experiment with different orientations to minimize the number of support structures required and maximize the surface area in contact with the build plate for better adhesion. Consider scaling your model to fit within the build volume of your 3D printer and to ensure that fine details are preserved without compromising print quality. Additionally, ensure that the model is centered and aligned properly on the build plate to avoid printing errors and ensure uniform adhesion.
Print Settings Configuration:
Configure print settings such as layer height, print speed, infill density, and temperature according to the requirements of your model and the capabilities of your 3D printer. Choose an appropriate layer height to balance print quality with printing time, considering factors such as surface finish and detail resolution. Adjust print speed and temperature settings to optimize print quality, adhesion, and material flow, taking into account the properties of the filament being used and the complexity of the model. Experiment with infill density to achieve the desired balance between strength and material usage, selecting higher densities for structural parts and lower densities for prototypes or decorative objects.
Print Preview and Simulation:
Before initiating the printing process, use print preview and simulation features available in slicing software to visualize the print path, identify potential issues, and verify that the model is prepared correctly. Review the layer-by-layer preview to ensure that support structures are generated as intended and that the model is sliced correctly without missing or overlapping layers. Use simulation tools to simulate the printing process and identify potential issues such as collisions, warping, or inadequate cooling that may occur during printing. Make any necessary adjustments to print settings or model orientation based on the results of the simulation to ensure a successful print.
8. Operating a 3D Printer:
Operating a 3D printer is an exciting and rewarding endeavor, allowing you to transform digital designs into tangible objects with precision and creativity. In this section, we delve into the essential steps and considerations involved in operating a 3D printer, from setup and calibration to monitoring and troubleshooting, ensuring a smooth and successful printing experience.
Setup and Calibration:
Begin by setting up your 3D printer in a well-ventilated and stable environment, ensuring that it is placed on a level surface and away from flammable materials or sources of heat. Follow the manufacturer’s instructions to assemble the printer, install the build plate, and load the filament into the extruder. Perform initial calibration procedures, such as leveling the build plate, adjusting the nozzle height, and calibrating the extruder steps/mm, to ensure proper operation and accurate printing.
Preparing the Print Bed:
Before initiating a print, prepare the print bed by applying an appropriate adhesive or surface treatment to promote adhesion and prevent warping. Depending on the type of printer and materials being used, this may involve applying adhesive sprays, tapes, or specialized build surfaces such as PEI or BuildTak. Ensure that the print bed is clean and free of debris or residue that may interfere with adhesion or cause print defects.
Loading Filament and Initiating Prints:
Load the filament into the extruder according to the manufacturer’s instructions, ensuring that it is fed smoothly and securely into the hot end assembly. Use the printer’s interface or control software to select the desired print file (G-code) and initiate the printing process. Monitor the printer during the initial stages of printing to ensure that filament is extruding properly and adhering to the print bed. Adjust print settings or stop the print if any issues arise, such as filament jams, bed adhesion problems, or extrusion inconsistencies.
Monitoring and Maintenance:
Throughout the printing process, monitor the printer for any signs of issues or anomalies, such as layer misalignment, filament skipping, or print defects. Keep an eye on temperature readings, print progress, and filament usage to ensure that the printer is operating within specified parameters. Perform routine maintenance tasks, such as cleaning the print bed, lubricating moving parts, and inspecting belts and motors for wear or damage, to keep the printer running smoothly and reliably.
Post-Processing and Finishing:
Once the print is complete, carefully remove the object from the print bed using a spatula or scraper, taking care not to damage the build surface or the object itself. Clean any support structures or residue from the print using appropriate tools and solvents, if necessary. Perform post-processing steps such as sanding, painting, or smoothing to achieve the desired surface finish and appearance. Store leftover filament in a dry, airtight container to prevent moisture absorption and maintain print quality for future use.
Troubleshooting and Optimization:
Inevitably, you may encounter issues or challenges during the printing process. Learn to troubleshoot common problems such as under-extrusion, layer shifting, or adhesion issues by adjusting print settings, inspecting hardware components, and consulting resources such as online forums, user manuals, and troubleshooting guides. Keep a record of successful prints, failed prints, and any adjustments made to print settings or hardware configurations to inform future printing endeavors and optimize your workflow.
9. Common Challenges and Troubleshooting:
As with any technological endeavor, 3D printing comes with its own set of challenges and potential pitfalls. From filament jams to print adhesion issues, understanding and troubleshooting these common problems is essential for achieving successful prints. In this section, we’ll explore some of the most frequent challenges encountered in 3D printing and provide tips for troubleshooting and resolving them effectively.
Filament Jamming:
Filament jams occur when the filament gets stuck in the extruder assembly, preventing smooth extrusion and disrupting the printing process. To troubleshoot filament jams, first, check for any obstructions or clogs in the nozzle or hot end assembly. Clear any debris or residue using a cleaning tool or needle, and ensure that the filament path is free from tangles or knots. Adjust the extrusion temperature and retraction settings to optimize filament flow and reduce the risk of jams in the future.
Poor Print Adhesion:
Poor adhesion between the printed object and the build plate can lead to warping, lifting, or failed prints. To improve print adhesion, ensure that the print bed is clean and properly leveled, and apply an appropriate adhesive or surface treatment to promote adhesion. Adjust print settings such as the initial layer height, print speed, and extrusion temperature to optimize adhesion and minimize the risk of detachment during printing. Experiment with different bed temperatures and adhesion methods to find the optimal combination for your printer and materials.
Layer Misalignment or Shifting:
Layer misalignment or shifting occurs when the layers of the print are not properly aligned, resulting in a skewed or distorted object. To troubleshoot layer misalignment, check for loose belts, worn pulleys, or mechanical issues that may be causing the printer to lose steps or skip layers. Tighten belts, lubricate moving parts, and ensure that the printer’s frame is stable and secure. Adjust print settings such as acceleration, jerk, and travel speed to reduce the risk of layer shifting and improve print quality.
Stringing and Oozing:
Stringing and oozing occur when excess filament is deposited between printed features, leading to unsightly strings or blobs on the finished object. To minimize stringing and oozing, adjust retraction settings to retract filament during non-printing moves, reducing the risk of excess material buildup. Fine-tune print temperature, cooling fan speed, and travel speed to optimize filament flow and minimize the likelihood of stringing or oozing between printed features. Experiment with different retraction distances and speeds to find the optimal settings for your printer and materials.
Print Warping and Curling:
Print warping and curling occur when the edges of the printed object lift or warp away from the build plate during printing, resulting in a distorted or uneven surface. To prevent warping and curling, ensure that the print bed is heated to the appropriate temperature for the filament being used and that the build plate is properly leveled. Apply an appropriate adhesive or surface treatment to promote adhesion and minimize the risk of detachment. Use a brim or raft to provide additional support and stability for large or thin-walled prints, and adjust print settings such as print speed and cooling fan speed to optimize layer adhesion and minimize thermal stress.
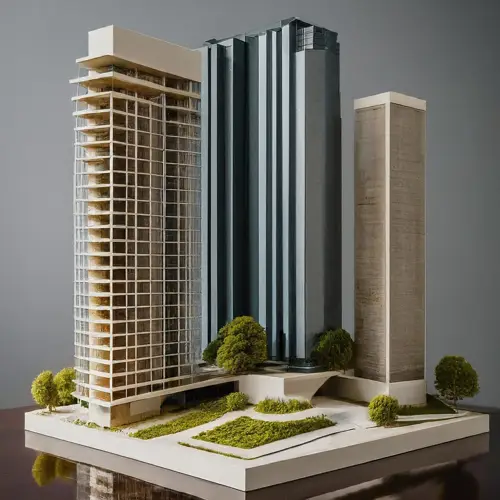
10. Applications of 3D Printing:
The transformative capabilities of 3D printing extend across a wide range of industries and disciplines, revolutionizing the way we design, manufacture, and interact with objects. From rapid prototyping and customized production to innovative medical treatments and sustainable construction, the applications of 3D printing are as diverse as they are impactful. In this section, we’ll explore some of the most prominent and promising applications of 3D printing across various fields.
Rapid Prototyping:
One of the earliest and most widely adopted applications of 3D printing is rapid prototyping, allowing designers and engineers to quickly iterate through multiple design iterations and test concepts before committing to mass production. 3D printing enables rapid turnaround times, reduced costs, and enhanced flexibility, making it an invaluable tool for accelerating product development cycles and bringing ideas to market faster.
Customized Manufacturing:
3D printing enables customized manufacturing at scale, allowing for the production of personalized products tailored to individual needs and preferences. From custom-fit prosthetics and orthotics to bespoke jewelry and footwear, 3D printing offers unparalleled freedom to create unique, one-of-a-kind objects that reflect the diversity of human anatomy, taste, and lifestyle.
Medical Applications:
In the field of medicine, 3D printing holds immense promise for revolutionizing patient care and treatment. From anatomical models and surgical guides to patient-specific implants and prosthetics, 3D printing enables precise and personalized solutions for a wide range of medical conditions and procedures. Additionally, bioprinting technologies are being developed to fabricate living tissues and organs for transplantation, regenerative medicine, and drug testing, offering hope for addressing the global organ shortage crisis.
Aerospace and Automotive:
In the aerospace and automotive industries, 3D printing is driving innovation and efficiency by enabling the production of lightweight, complex components with optimized performance characteristics. From engine parts and turbine blades to interior panels and prototypes, 3D printing offers designers unprecedented freedom to explore novel geometries and materials, resulting in improved fuel efficiency, reduced emissions, and enhanced safety.
Architecture and Construction:
In architecture and construction, 3D printing is revolutionizing the way buildings and infrastructure are designed and fabricated. Large-scale 3D printers can construct entire structures, such as houses, bridges, and pavilions, using a variety of materials ranging from concrete and clay to recycled plastics and biomaterials. 3D printing enables rapid construction, reduced waste, and enhanced design flexibility, paving the way for sustainable, cost-effective solutions to housing and infrastructure challenges around the world.
Education and Research:
In education and research, 3D printing serves as a powerful tool for hands-on learning, experimentation, and innovation. From classrooms and makerspaces to research labs and universities, 3D printers provide students and researchers with the opportunity to explore concepts in science, technology, engineering, and mathematics (STEM) in a tangible and interactive way. 3D printing fosters creativity, critical thinking, and problem-solving skills, preparing the next generation of innovators and entrepreneurs for success in a rapidly evolving world.
Consumer Products and Fashion:
In the realm of consumer products and fashion, 3D printing is pushing the boundaries of creativity and customization, enabling designers to create unique, avant-garde pieces that defy traditional manufacturing constraints. From fashion accessories and wearable tech to home decor and consumer electronics, 3D printing offers consumers the opportunity to express their individuality and style through personalized, made-to-order products that reflect their personality and taste.
Environmental Conservation:
In the fight against climate change and environmental degradation, 3D printing holds promise for promoting sustainability and resource conservation. By enabling localized production, on-demand manufacturing, and the use of recycled and biodegradable materials, 3D printing can reduce the carbon footprint associated with traditional manufacturing processes and supply chains. Additionally, 3D printing can be used to fabricate low-cost, eco-friendly solutions for environmental monitoring, remediation, and conservation efforts, such as biodegradable sensors and water filtration systems.
11. Future Trends in 3D Printing:
As 3D printing continues to evolve and mature, exciting new trends and advancements are emerging that promise to revolutionize the way we design, manufacture, and interact with objects. From breakthrough materials and technologies to novel applications and industries, the future of 3D printing is filled with possibilities. In this section, we’ll explore some of the most promising trends and developments shaping the future of additive manufacturing.
Advanced Materials:
One of the most significant trends in 3D printing is the development of advanced materials that expand the capabilities and applications of additive manufacturing. Researchers are exploring a wide range of materials, including metals, ceramics, composites, and biomaterials, with enhanced properties such as strength, conductivity, and biocompatibility. These materials enable the fabrication of functional prototypes, end-use parts, and custom implants with unprecedented precision and performance, opening up new opportunities in industries such as aerospace, healthcare, and automotive.
Multi-Material and Multi-Color Printing:
Advancements in multi-material and multi-color printing technologies are enabling designers to create more complex, detailed, and visually appealing objects than ever before. With the ability to print with multiple materials and colors in a single process, designers can achieve intricate color gradients, textures, and material transitions that were previously impossible with traditional manufacturing methods. This trend is particularly relevant in applications such as consumer products, fashion, and art, where aesthetics and customization are paramount.
Continuous Printing and Large-Scale Additive Manufacturing:
Continuous printing and large-scale additive manufacturing are revolutionizing the way we build structures and infrastructure, from houses and bridges to spacecraft and aircraft components. By utilizing robotic arms, gantry systems, and other automated technologies, manufacturers can fabricate large-scale objects with unprecedented speed, precision, and cost-effectiveness. This trend is driving innovation in construction, aerospace, and automotive industries, offering sustainable, scalable solutions to complex engineering challenges.
Digital Twinning and Simulation:
Digital twinning and simulation technologies are enabling designers and engineers to create virtual replicas of physical objects and systems, allowing for real-time monitoring, analysis, and optimization throughout the product lifecycle. By integrating 3D printing with digital twin models, manufacturers can simulate and validate designs before physical prototyping, reducing time-to-market and minimizing costly errors. This trend is transforming industries such as healthcare, where patient-specific models and simulations enable personalized treatments and surgical planning with unprecedented accuracy and efficacy.
Additive Manufacturing in Space:
As humanity ventures further into space exploration and colonization, 3D printing is playing an increasingly important role in enabling sustainable, self-sufficient habitats and infrastructure in space. Additive manufacturing technologies are being developed to fabricate tools, spare parts, and even habitats using locally sourced materials, reducing the need for costly and logistically challenging supply missions from Earth. This trend has profound implications for the future of space exploration and colonization, offering a pathway to sustainable, long-term human presence beyond Earth.
Machine Learning and Artificial Intelligence:
Machine learning and artificial intelligence are being leveraged to optimize and automate various aspects of the 3D printing process, from design optimization and print parameter selection to quality control and defect detection. By analyzing vast amounts of data and identifying patterns and trends, AI-powered systems can enhance efficiency, accuracy, and reliability in additive manufacturing, leading to faster iteration cycles, higher-quality prints, and reduced waste. This trend is driving innovation across industries and accelerating the adoption of 3D printing as a mainstream manufacturing technology.
Bioprinting and Tissue Engineering:
In the field of healthcare, bioprinting and tissue engineering are opening up new frontiers in regenerative medicine, drug discovery, and personalized healthcare. Researchers are developing bioprinting technologies capable of fabricating living tissues and organs using patient-specific cells and biomaterials, offering potential solutions to organ shortage and transplant rejection. This trend holds promise for revolutionizing patient care and treatment, enabling personalized therapies and interventions that were previously unimaginable.
12. Conclusion:
In conclusion, 3D printing stands at the forefront of a transformative revolution in manufacturing and design. From its humble beginnings as a niche technology to its current status as a mainstream manufacturing tool, 3D printing has evolved rapidly, unlocking new possibilities and reshaping industries across the globe. In this blog, we’ve explored the fundamentals of 3D printing, from its underlying principles and technologies to its applications and future trends.
Throughout its journey, 3D printing has democratized manufacturing, empowering individuals, entrepreneurs, and businesses of all sizes to bring their ideas to life with unprecedented speed, precision, and creativity. Rapid prototyping, customized manufacturing, and on-demand production have become the norm, enabling faster innovation cycles, reduced time-to-market, and enhanced product customization.
Moreover, 3D printing has revolutionized industries ranging from healthcare and aerospace to fashion and construction, offering novel solutions to complex challenges and driving unprecedented levels of innovation and efficiency. Breakthrough materials, multi-material printing, and large-scale additive manufacturing are pushing the boundaries of what’s possible, enabling the creation of structures, products, and systems that were previously unimaginable.
Looking ahead, the future of 3D printing holds immense promise, with advancements in materials, technologies, and applications poised to unlock new opportunities and reshape industries in profound and transformative ways. From bioprinting and tissue engineering to additive manufacturing in space, the possibilities are endless, offering a glimpse into a future where creativity, sustainability, and innovation reign supreme.
As we embark on this journey into the future of 3D printing, let us embrace the opportunities and challenges that lie ahead, harnessing the power of technology to create a more sustainable, efficient, and inclusive world for generations to come. With creativity, collaboration, and dedication, we can continue to push the boundaries of what’s possible and unlock the full potential of additive manufacturing to shape a brighter tomorrow.